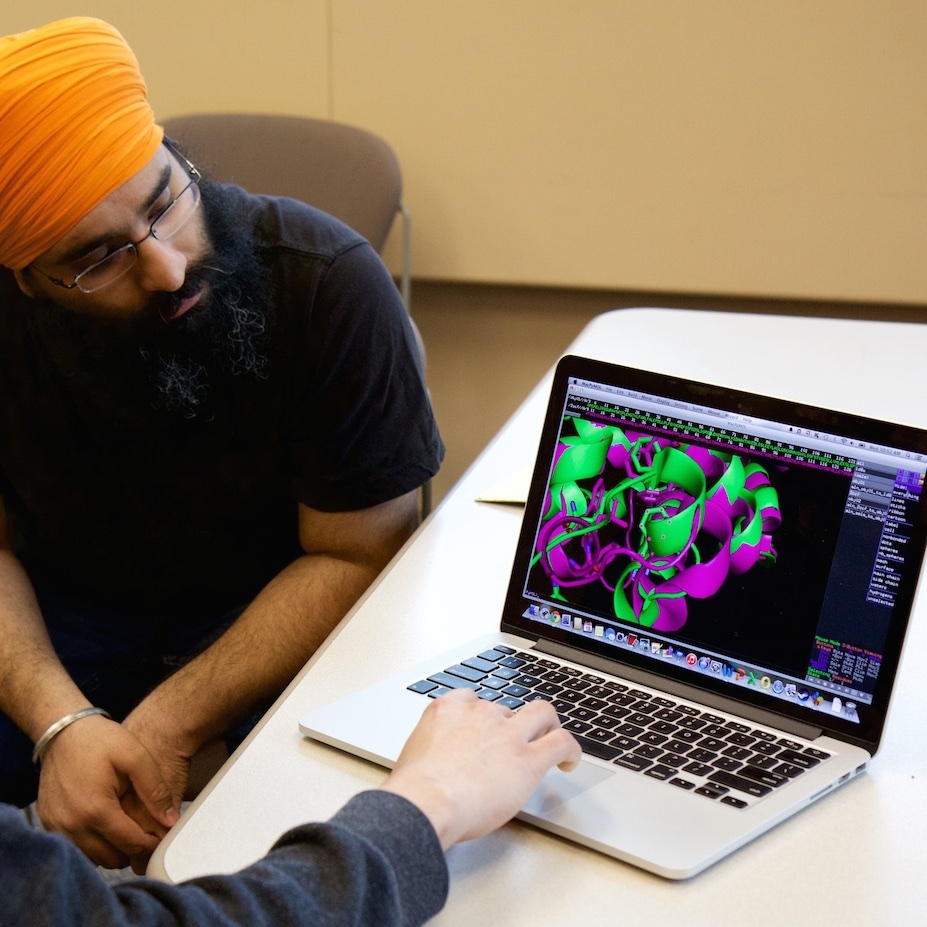
We’re known for our strengths in the life sciences. Collaborations in LAS between biological and physical sciences have made a significant impact on drug development, plant and animal health, cancer research, and more. Now, our researchers are working to engineer a new protein that could protect our cells against heart attack and stroke.
If you have heart complications, you may be taking nitrates to alleviate your condition. But no one is really sure why.
The effect is understood – nitrates dilate arteries and veins to reduce stress on the heart muscle, thus increasing the amount of oxygen available to the heart – but new research is shedding light on how. The effects of nitrates may be linked to the activity of a small molecule called nitric oxide, that could lead to big discoveries in pharmaceutical exploration.
Nitrates and nitrites (commonly found in food preservatives and fertilizers, respectively) may create nitric oxide when they are exposed to hemoglobin. The resulting nitric oxide can be beneficial – helping blood vessels open up to deliver blood – but it can be damaging if there isn’t enough oxygen present, resulting in heart attacks and strokes.
“The relationship between compounds such as nitrite and nitrate in animals is traditionally thought of as kind of boring because we don’t do much with either of them,” Mark Hargrove, professor in the Roy J. Carver Department of Biochemistry, Biophysics and Molecular Biology (BBMB), said. “But new research over the past 10 years has suggested that there might be more going on than we once thought.”
Researchers in the College of Liberal Arts and Sciences are trying to engineer a protein that would protect human cells from injury when they are starved for oxygen. To do this, they’re looking in an unexpected place: plants.
“We’ve been studying protein structures and their chemical functions for a long time,” Hargrove said. “And we’ve found plant proteins that do some chemistry which could be therapeutically beneficial that animal proteins do not.”
To engineer the protein, Hargrove paired up with Amy Andreotti, a Carver Charitable Trust professor in BBMB, who uses her lab to replace one small piece of an animal protein with a piece of plant protein. Piece by piece, her team watches the activity and reactions of each step with the goal of designing a molecule that will mitigate the damage caused by nitric acid during a stroke or heart attack.
“The plant versions of these hemoglobins do this very well,” Hargrove said. “The human versions do not. We want to design the plant activity in the human protein scaffolding.”
It’s innovative, exciting work that’s high risk, but if Hargrove’s hypothesis is correct, it would be groundbreaking.
The building blocks of an experiment
In Andreotti’s lab, two graduate students, Jagannathan Alagurajan (from the Andreotti lab) and Navjot Singh (from the Hargrove lab), are monitoring proteins in a “glove box” – an oxygen-free, sealed container built to mimic the conditions of our blood. The experiments were set the night before, and Alagurajan and Singh now use spectrometry to see how each protein absorbs light, which helps them monitor oxygen and absorption levels in each protein.
Looking at a chart on a computer screen, they point to lines that represent what each protein would look like in a human body. Different lines in shades of red represent each newly engineered protein. If they see potential in the biochemistry of one of the proteins, they analyze it in detail in a separate computer program, looking at the whole protein to identify characteristics that aren’t present in a plant protein.
Alagurajan describes this process as being similar to building with Legos.
“Plant proteins are different, structurally,” he said. “So we take pieces of the plant protein and place it in the human protein to see if that gives us better activity or better reactions. If it doesn’t work, we switch to a different Lego piece and keep repeating that until we find one Lego piece that will work to move from one scaffold to another.”
The team looks at several protein pieces at once – running multiple experiments side-by-side. The whole process is fast, and takes about a month.
Testing a hypothesis
Once Hargrove and Andreotti’s team have designed a molecule they think has the properties needed to act as a piece of a plant protein, they move it to another lab to introduce it back into an animal system.
In this lab, Jo Anne Powell-Coffman introduces the new proteins to the cells of C. elegans (a tiny roundworm commonly used in genetics research). Then, she watches the stress response in the worms.
“We have published several papers on how C. elegans adapt to different oxygen levels,” Powell-Coffman, professor and chair of the Department of Genetics, Development and Cell Biology, said. “We’ve found that some of the cellular networks that facilitate the adaptations needed for animals to survive low oxygen are the same in these tiny worms as in humans. As we learn what helps the worm survive, we learn what could help humans survive.”
Powell-Coffman has just begun this stage of the research project, which was provided seed funding from LAS as part of a Signature Research Initiative grant in 2013. Hargrove and Andreotti spearheaded the first leg of the project, which will continue through 2016.
C. elegans is a simple animal that researchers understand well. They allow scientists to conduct experiments quickly and on a small budget. Even if it were just as easy to introduce the new proteins to a mouse or a human, Powell-Coffman said C. elegans would still be used, because they don’t have a complex immune system. If new plant proteins were introduced in humans first, our immune systems would immediately recognize it as a foreign protein and attack it.
Hargrove’s understanding of how plant proteins work is what makes this project so unique. Researchers in his field haven’t thought of plant globins as therapeutic agents – usually because they are focused on protecting plant biodiversity, feeding the world, or other agricultural-related efforts. All great things, of course. But translating his understanding of how plant proteins work to the medical field illustrates a deeper level of thinking that is not a common avenue in plant biology.
Powell-Coffman is excited about the trails this will blaze.
“If we get the initial result that we’re looking for, then the riskiest part is done,” she said. Next steps would include testing on larger animals, and someday, possibly, protecting our cells from oxygen starvation.